Exploring Obesity: From the Depths of the Brain to the Far Pacific
Videos are generally available for preview to non-members as short clips. Limited full-length titles are also available. Log In to view the full length title.
Browse Full-length Non-member Titles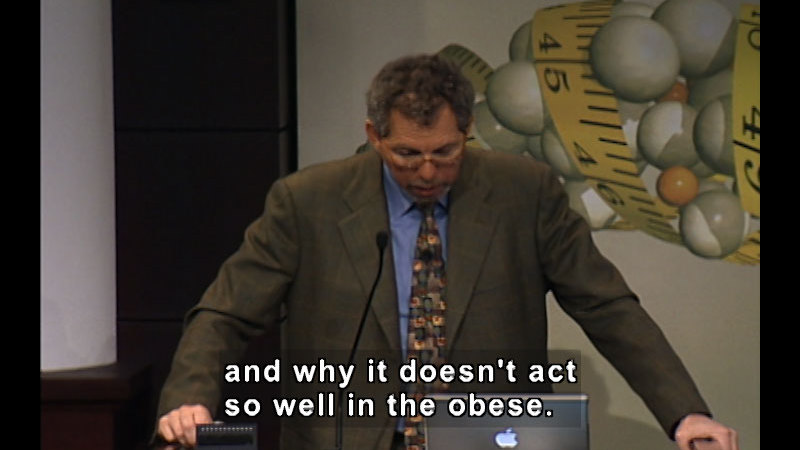
Dr. Jeffrey Friedman shows how leptin rewires neural circuits, and how population studies may identify obesity genes. Part of the 2004 Howard Hughes Holiday Lecture Series
Media Details
Runtime: 58 minutes 30 seconds
- Topic: Science, Sports and Recreation
- Subtopic: Biology, Physical Fitness, Physiology, Science Experiments
- Grade/Interest Level: 11 - 12
- Standards:
- Release Year: 2005
- Producer/Distributor: Howard Hughes Medical Institute
- Series: Holiday Lectures On Science
- Report a Problem
Available Resources
Request a DVD
Web Page
Members Only - Apply Now
Related Media
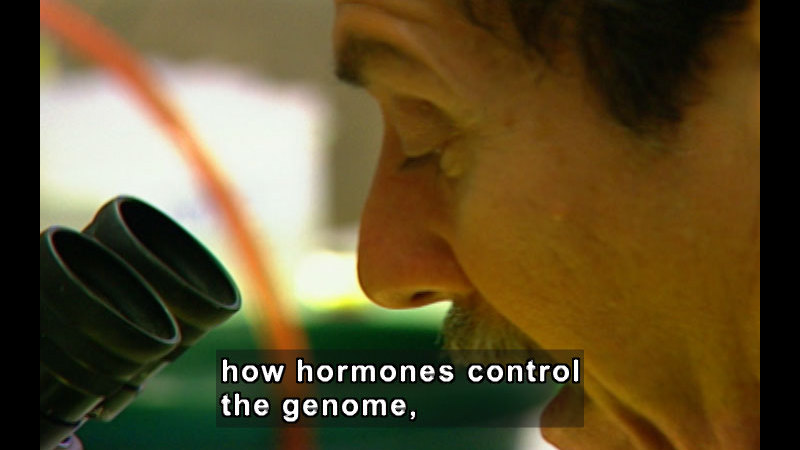
Holiday Lectures On Science
Season 2004 / Ep 1
58 minutes 30 seconds
Grade Level: 11 - 12
Holiday Lectures On Science
Season 2004 / Ep 1
58 minutes 30 seconds
Grade Level: 11 - 12
Understanding Fat: Syndrome X and Beyond
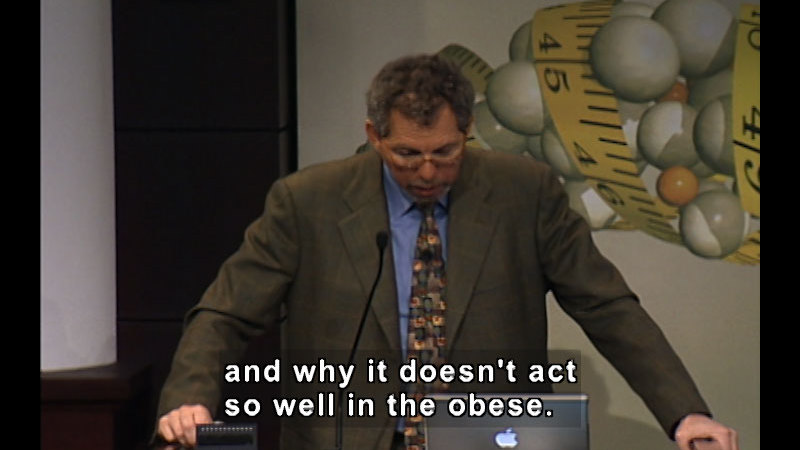
Holiday Lectures On Science
Season 2004 / Ep 2
58 minutes 30 seconds
Grade Level: 11 - 12
Holiday Lectures On Science
Season 2004 / Ep 2
58 minutes 30 seconds
Grade Level: 11 - 12
Exploring Obesity: From the Depths of the Brain to the Far Pacific
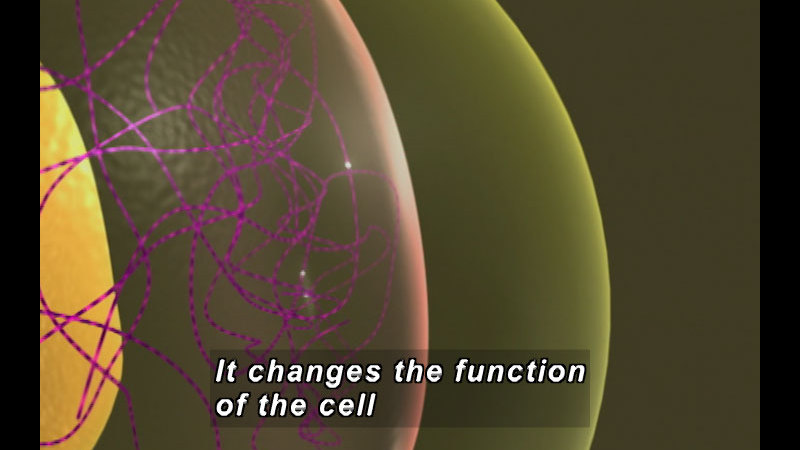
Holiday Lectures On Science
Season 2004 / Ep 3
58 minutes 28 seconds
Grade Level: 11 - 12
Holiday Lectures On Science
Season 2004 / Ep 3
58 minutes 28 seconds
Grade Level: 11 - 12
Balancing the Fat Equation
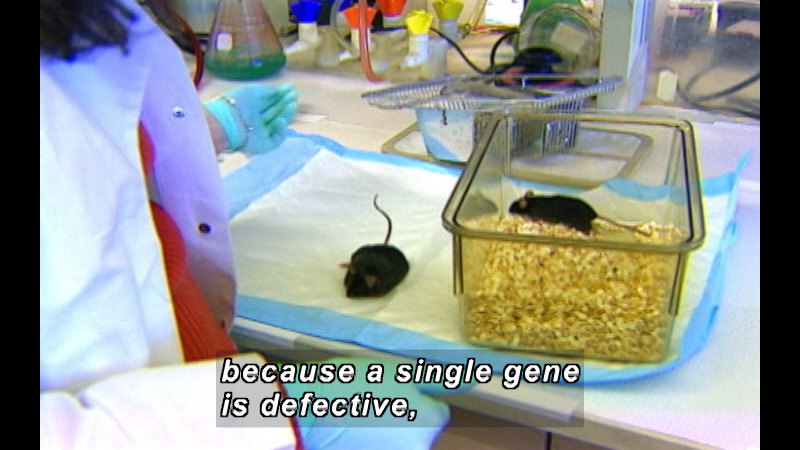
Holiday Lectures On Science
Season 2004 / Ep 4
58 minutes 30 seconds
Grade Level: 11 - 12
Holiday Lectures On Science
Season 2004 / Ep 4
58 minutes 30 seconds
Grade Level: 11 - 12
Deconstructing Obesity